Überblick über Elektronenstrahl-Schmelzanlage
Electron beam melting (EBM) is an additive manufacturing technology used to fuse metal powders into fully dense parts layer-by-layer using a high power electron beam under vacuum conditions. EBM machines offer unmatched build speeds and mechanical properties unachievable with other metal 3D printing methods.
Key attributes of EBM technology include:
Table 1: Overview of Electron Beam Melting Technology
Attribut | Beschreibung |
---|---|
Wärmequelle | High intensity electron beam |
Environment | High vacuum |
Rohstoff | Metal powder bed |
Beam Control | Electromagnetic lenses and coils |
Build Mode | Layer-by-layer metal powder fusion |
Anwendungen | Aerospace, medical, automotive, tooling |
By leveraging precise beam focusing and rapid scanning, EBM fuses conductive materials like titanium, nickel alloys, tool steels, and refractory metals into fully dense components with superior properties exceeded only by wrought products.
The controlled vacuum environment prevents contamination while the intelligent energy delivery and high preheat temperatures minimize residual stresses leading to warp or cracks.
Understanding these core principles helps illustrate why EBM delivers exceptional mechanical performance tailor-made for the most demanding industry applications.
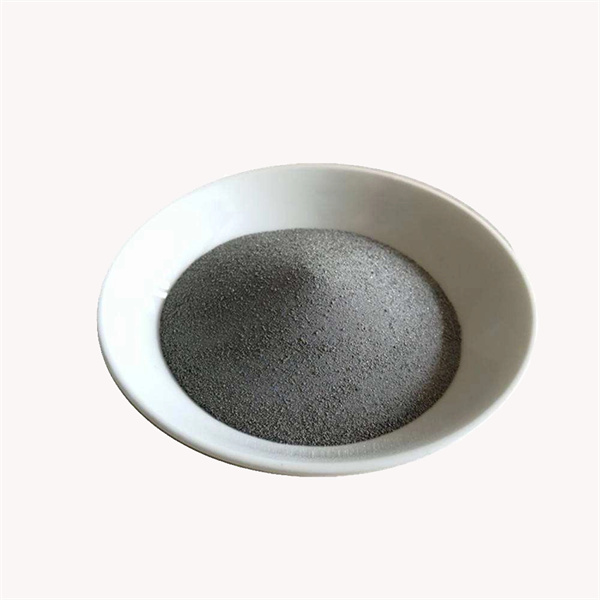
Types of Electron Beam Melting Systems
There are several categories of EBM systems on the market offering build volumes, beam power levels, and production capacities catering to different industry needs.
Table 2: Types of Electron Beam Melting Systems
Machine Class | Größe bauen | Strahlleistung | Typische Anwendungen |
---|---|---|---|
Small platforms | 150 mm cubes | 3-4 kW | Dental copings, medical devices |
Standard platforms | 200 x 200 x 350 mm | 6-8 kW | Aerospace components, tooling |
Mid-range platforms | 400 x 400 x 400 mm | 14-16 kW | Automotive parts, larger aerospace components |
Large platforms | 800 x 800 x 500 mm | 30-60 kW | Structural brackets, turbine blades |
Larger machines allow bigger parts for industries like aerospace or automotive to optimize assemblies. Smaller, lower power systems target high value components in the dental and wider medical space.
Most EBM providers now offer modular architectures allowing capacity, build volume, and beam power scalability to match rising production demands over time.
Fundamentals of Electron Beam Melting Process
The core subsystems and processing steps involved in EBM additive manufacturing encompass:
Table 3: Overview of Electron Beam Melting Fundamentals
Bühne | Funktion | Wichtige Komponenten |
---|---|---|
1. Powder handling | Distribute layers of fresh material | Powder hoppers and rakes |
2. Beam generation | Create and accelerate electron beam | Tungsten filament cathode, anode voltage |
3. Beam focusing | Electromagnets converge beam | Magnetic coil lenses |
4. Beam deflection | Direct focused beam location | Deflection coils |
5. Vacuum system | Ensure contaminant-free environment | Pumps, valves, sensors |
6. Control system | Coordinate and monitor all functions | Computer, software, sensors |
Integrated operation of these subsystems lets EBM efficiently build parts layer-by-layer from metal powder feedstock:
- Rapid beam deflection and scanning fuses material precisely with exceptional speed
- Vacuum environment removes gases preventing contamination
- Automatic powder distribution ensures high density
- Feedback sensors provide dimensional accuracy
- Robust controls sequence the entire build process
This combination of a pure metal powder consumable with a high intensity beam heat source in a vacuum production environment facilitates previously impossible materials performance.
Grasping these foundational principles assists buyers in selecting the optimum EBM system meeting their manufacturing productivity, quality, and application requirements.
Key Specifications of Elektronenstrahl-Schmelzanlage
When purchasing EBM equipment for metal additive manufacturing, there are numerous performance-driving specifications buyers must evaluate based on their production objectives and facility constraints.
Table 4: Key Electron Beam Melting Machine Specifications
Parameter | Typischer Bereich | Bedeutung |
---|---|---|
Strahlleistung | 3-60 kW | Build rate, maximum part size |
Strahlgeschwindigkeit | Up to 8 m/s | Productivity, layer times |
Punktgröße | 50-200 μm | Resolution, fine feature definition |
Strahlstrom | 1-50 mA | Material compatibility, tuning optimization |
Beschleunigungsspannung | 30-150 kV | Melt pool depth, leftover powder |
Vakuum | 5 x 10-5 mbar | Purity, material integrity |
Powder layer thickness | 50-200 μm | Vertical resolution, final density |
Factors like beam power, scanning speeds, minimum feature sizes, and powder layer thickness dictate suitable equipment selection aligned with productivity objectives and application requirements.
Other key considerations include:
- Steuerungssoftware – Adaptive build set up tools, automation, data analytics/monitoring capabilities
- Materials palette – Number of pre-qualified materials indicating application range
- Ancillary equipment – Supplementary powder handling tools, post-processing, heat treatment ovens
- Dienstleistungen – Maintenance contracts, application optimization assistance, operator training, machine transport
Evaluating specifications against current and future expectations facilitates smart investments in EBM capacity.
Economics of Electron Beam Melting Adoption
Aside from equipment acquisition costs averaging $800,000 to $2.5 million, manufacturing organizations must model the entire production economics from bringing EBM in-house.
Table 5: Summary of EBM Processing Economics
Cost Element | Bereich |
---|---|
Machine platform | $800,000 to $2,500,000 |
Facility infrastructure | $100,000 to $500,000 |
Installation services | $50,000 to $250,000 |
Supplementary powder handling tools | $50,000 to $150,000 |
Annual materials usage | $100,000 to $800,000 |
Consumables/replacement parts | $20,000 to $100,000 |
Labor (operators, engineers) | 1 to 3 technicians per system |
Energieverbrauch | $15,000 to $50,000 |
Maintenance contracts | $50,000 to $150,000 |
Aside from equipment acquisition falling between $800,000 to over $2 million for industrial platforms, other variables influencing operating costs and profitability encompass:
- Material usage – Metal powder contributes up to 30% towards costs per part
- Arbeit – Staffing requirements driven by manual vs automated post-processing needs
- Facility – Installation services, safety, and utility expenses add up
- Wartung – Preventative upkeep is vital for production throughput and quality
- Optimization – Balancing productivity versus defect rates and manual interventions
Analyzing these factors before acquiring EBM capacity facilitates realistic business planning. Accurate cost modeling and production scenario analysis improves visibility into risks and profitability outlooks.
Popular Materials for EBM
Thanks to a tightly controlled vacuum environment combined with high beam intensities, EBM uniquely facilitates processing reactive, refractory, and custom alloys otherwise difficult to manufacture using conventional means.
**Table 6: Common Alloy Systems Leveraging EBM Benefits **
Material Class | Beispiel Legierungen | Anwendungen |
---|---|---|
Titan-Legierungen | Ti-6Al-4V, Ti-6Al-4V ELI | Aerospace airframes and engines |
Nickel-Superlegierungen | Inconel 718, Inconel 625 | Turbinenschaufeln, Raketendüsen |
Werkzeugstähle | Maraging 300, H13 | Injection molds, tooling plates |
Kobalt-Chrom-Legierungen | CoCrMo, CoCrW | Medical and dental implants |
Hochschmelzende Metalle | Tantalum, Tungsten | High temperature furnace elements, shielding |
The most popular alloy systems for EBM remain titanium alloys for structural components, nickel superalloys for extreme environments, and medical grade cobalt chrome formulations.
However, EBM also unlocks innovations utilizing reactive metals like aluminum or niobium rarely processable otherwise. Combined with flexible powder mixing options, research bureaus exploit EBM benefits for designing new alloys compositions tailored around specific property requirements.
Benefits of Electron Beam Melting
Aside from extremely rapid build speeds unmatched by other powder bed fusion techniques, EBM offers additional technical and economic advantages making it the ideal process for critical commercial and defense applications.
**Table 7: Primary Benefits of Electron Beam Melting **
Nutzen Sie | Beschreibung |
---|---|
High deposition rates | Up to 10x faster builds than laser-based systems |
Exceptional material properties | Improved beyond cast or wrought alternatives |
High production density | Approaches 100% thanks to high beam energy in vacuum environment |
Very low residual stress | 70-90% less distortion reduces machining allowance needs |
Exceptional repeatability | Tight tolerances and mechanicals from automated building |
Gestaltungsfreiheit | Internal channels, bionic structures, weight reduction |
Teilweise Konsolidierung | Assemblies combined into single components |
Specific examples where EBM delivers value encompass:
Produktivität
- Manufacturing hip implant assemblies 5x faster by leveraging larger build volumes produce more units simultaneously
- Consolidating aerospace landing gear component inventories from 30 to 2 parts via EBM optimization efforts
Leistung
- Offering better fatigue resistance in cobalt chrome dental copings over casting
- Achieving cleaner Inconel 718 microstructures completely free of porosity defects from traditional nickel superalloy castings
Qualität
- Ensuring zero internal stresses in Ti-6Al-4V medical components thanks to high pre-heat, reducing scrap rates
- Preventing contamination defects in reactive Ta and Nb alloys by leveraging vacuum processing environment
Thanks to faster builds and exceptional materials properties impossible with other metal AM or conventional techniques, EBM is the foremost solution for production applications requiring the highest levels of mechanical performance.
Überblick über Elektronenstrahl-Schmelzanlage Anbieter
A variety of established industrial manufacturers and specialized new entrants provide electron beam melting solutions scalable from research to high volume production across aerospace, medical, automotive, and industrial sectors.
Table 8: Leading Electron Beam Melting System Manufacturers
Anbieter | Einzelheiten | Target Segments |
---|---|---|
GE-Zusatzstoff | Pioneered EBM technology | Aerospace, medical, automotive |
Sciaky | Largest envelope size | Strukturen für die Luft- und Raumfahrt |
Wayland-Zusatzstoff | Budget metal AM platforms | Small machine shops |
JEOL | Research grade EBM systems | Universities |
Nanodimension | Multi-material capabilities | Electronics, defense |
Industry leader Arcam EBM, now part of GE Additive, established early leadership thanks to patented solutions and continues dominating the established medical implant and aerospace categories.
Meanwhile, new entrants like Wayland aim to expand adoption by targeting small and medium manufacturers with economical starting platforms.
Collaborations on materials, part qualification, and machine optimization between producers, researchers, and end-user groups will ultimately expand EBM penetration across further critical applications.
Future Outlook for Electron Beam Melting Adoption
Propelled by superior production speed capabilities plus exceptional mechanical properties impossible with other metal additive or conventional process, EBM adoption appears positioned for massive expansion across aerospace, medical device, automotive, and industrial categories over the next 5-7 years.
Broader awareness of EBM benefits beyond prototyping into full-scale production is expected to drive equipment investments as organizations leverage 3D printing to reshape supply chains.
Larger build envelopes now commercially accessible also enable consolidation of assemblies into fewer components, further optimizing inventory logistics and lead times.
However, declines in system costs combined with increased materials availability must continue improving smaller manufacturers’ access to EBM technology. Streamlining ancillary powder handling tools and post-processing workflows will also simplify adoption.
Overall, EBM sustains strong momentum to penetrate an increasingly wider range of production applications thanks to unmatched deposition rates and exceptional resultant material properties relative to alternate metal additive or legacy fabrication processes.
FAQ
Q: What facility infrastructure is needed to support EBM?
A: Expect 500+ sq. ft for the machine itself, with more space for powder handling stations and post-processing. Concrete floor reinforcement for 12,000+ lb equipment loads is typical.
Q: How many operators per EBM machine are required?
A: One technician can support several EBM units depending on automation level and production volumes. Additional staff handles powder operations, post-processing tasks, maintenance, and engineering.
Q: What materials cannot be processed with EBM technology?
A: Non-conductive polymers cannot be processed with electron beams. But EBM accommodates practically any conductive metal alloy system otherwise manufacturable.
Q: What safety risks are associated with EBM technology?
A: High power electron beam voltages pose arc flash risks requiring proper enclosures and controls. Reactive metal powder exposure also requires protocols for fire and inhalation dangers necessitating protective equipment and training.
Q: Does EBM require any secondary heat treatment?
A: Certain alloys benefit from heat treatment to further enhance microstructures and tailor mechanical properties. However, the rapid solidification cycles and high pre-heat temperatures intrinsic to the EBM process typically eliminate these post-processing steps.